The following information on tick paralysis in animals is provided for veterinarians.
Tick paralysis caused by Ixodes holocyclus is a major veterinary problem in Australia and it has been estimated that at least 10,000 dogs and cats are presented to veterinarians for treatment each year (Stone and JH, 1987). A wide range of other domesticated animal species are also affected including horses, cattle, sheep and poultry. Dogs and cats are perhaps the most important species affected in Australia for the veterinary profession. The diagnosis and treatment of tick paralysis cases, preventative acaricidal preparations provides a significant and seasonal workload for veterinarians. Although the life cycle of the tick has been understood for many decades, the elusive molecular nature of the toxin causing the neurological signs has been inadequately described until recently (Rodriguez-Valle et al., 2018; Thurn et al., 1992). Whilst Ixodes holocyclus is recognised as the main cause of tick paralysis in Australia, a morphologically similar and closely related species Ixodes cornuatus, is also capable of causing a similarly potentially fatal paralysis.
The scope of this review encompasses new research and the specific issues with tick paralysis in dogs and cats in Australia, and expands upon a previous broader review concerning all domestic animal species (Padula, 2016). Whilst there are other ticks found outside of Australia (Malik and Farrow, 1991) that can cause clinically significant paralysis in dogs and cats, they have not been included in this review. Although humans can be affected with all stages of the life cycle of the paralysis tick and suffer a profound and potentially fatal paralysis particularly in young children (Hamilton, 1940; Pearn, 1977), this material is outside the scope of this review. The Australian veterinarian Sir Ian Clunies Ross has made a major contribution to understanding the biology of the paralysis tick and developed the first canine tick antiserum (TAS); much of his early work is recognised in this review (Ross, 1935).
2.1 History
Tick paralysis has long been a problem on the east coast of Australia, first documented in 1884; although it had been recognised since the early settlement of the continent (Dodd, 1921). Experimental studies to better understand the biology of the tick and its ensuing pathophysiology were first begun in the 1920’s (Dodd, 1921; Ross, 1926, 1927a). Sir Ian Clunies Ross experimentally engorged ticks on dogs and was able to demonstrate that paralysis did not occur until 3 to 5 days after attachment, and sometimes up to 13 days. Ross also found that the toxin was likely to be produced from the tick salivary glands because injection of homogenised glands into normal dogs produced similar clinical signs (Ross, 1926).
Immunity was observed in dogs that had recovered from tick paralysis and Ross proposed that a hyperimmune serum made in dogs might be the only effective treatment but that its production “…would be difficult and costly…” (Ross, 1927b). Ross subsequently produced a hyperimmune serum and documented its therapeutic use in over 100 clinical cases of naturally occurring tick paralysis in dogs (Ross, 1935). Ross reported a 75% recovery rate with the serum and commented that veterinary practitioners at that time generally had a 10-60% recovery rate without the serum (Ross, 1935). Ross used a simple mouse protection bioassay to measure serum neutralising potency and demonstrated that his canine TAS could protect a mouse from 20-40 lethal doses of engorged tick extract (Ross, 1935). Whilst the tick serum proved useful, some veterinary practitioners still experienced deaths leading to the conclusion the serum was most useful only in the early stages of the disease process following tick removal (Hindmarsh and Pursell, 1935). Commercial production of the canine TAS first began in the 1930s at the Commonwealth Serum Laboratories using the methods developed by Ross (Oxer, 1948; Ross, 1935).
2.2 Life cycle of Ixodes holocyclus
Knowledge of the life cycle of Ixodes holocyclus has been progressively obtained using both laboratory colonies of ticks, field data from various infested animal species and the environment (Ross, 1924). Ixodes holocyclus has a life cycle similar to other members of the Ixodes tick family. It is a three-host tick, engorging on a blood meal and then dropping off its warm-blooded host between each successive stage of the life cycle (Figures 1-4). The life cycle is comprised of four stages: egg, larva, nymph and adult. The engorgement period for each feeding stage can last up to 14 days and is dependent upon environmental temperature and humidity. Large numbers of larvae, nymphs, and adults (females) may be found simultaneously on the same individual. Larvae and nymphs drop off the host when engorged and undergo a moult on the ground into the next stage of the lifecycle. When conditions are favourable the ticks begin to ‘quest’, actively seeking a host, attracted by carbon dioxide, heat and movement (Hall-Mendelin et al., 2011a). Engorged and sexually mature adult females detach and lay 2,000-3,000 eggs into moist litter. Males also seek warm blooded hosts but rarely take a blood meal from the host; instead they mate on the host and feed on the haemolymph of engorging female ticks by piercing their cuticle on their ventral side (Ross, 1924). The development from egg to adult takes around one year. However, cold conditions lengthen the developmental period. Dryness affects all stages adversely and this limits ticks to a habitat with a high relative humidity (>85%). The eggs hatch in 40-60 days during the summer months in moist leaf litter. The emerging larva can survive for weeks without attaching to a host. They appear mainly in summer and autumn (February to May). Nymphs can also survive without a host for long periods. They appear predominantly in autumn and winter (March to September) followed by adults with numbers peaking in late spring to early summer (September through to December) (Doube, 1975).
The bandicoot is an important intermediate host in Australia for Ixodes holocyclus and is arguably not the only ‘primary host’ of the tick (Lydecker et al., 2015). Bandicoots have been found with more than 60 adult ticks attached without clinical signs of tick paralysis (Jones, 1991). These sorts of observations have created conjecture as to whether the bandicoot has innate natural immunity or that it acquires protective immunity through chronic exposure; development of immunity through exposure appears the favourable hypothesis (Stone and Wright, 1981). The two significant bandicoot species are the short nose bandicoot found in eastern Queensland and north east NSW, and the long nose bandicoot found in eastern Queensland, eastern NSW and Victoria (Doube, 1975; Ross, 1924). Many other animal species can also function as host and provide the necessary blood meal, including the domestic animals such as dogs, cats, cows, horses, sheep and poultry (Ilkiw, 1983).
2.3 Distribution of I. holocyclus and I. cornuatus in Australia
Tick paralysis in Australia in animals is primarily caused by Ixodes holocyclus although a morphologically similar species – Ixodes cornuatus – is responsible for a lesser proportion of cases. Distinction between these two Ixodid species has been possible by careful morphological examination and more recently the key anatomical differences have been better defined, aiding their morphological differentiation (Barker and Walker, 2014; Kwak, 2017) (Table 1). Ixodes cornuatus has been associated with clinical signs of paralysis in animals (Beveridge and Coleman, 2004). This species of tick is found in south eastern Australia, including Tasmania where it appears to have been mistaken as Ixodes holocyclus (Ryan, 1952) where Ixodes holocyclus is not currently found (Jackson et al., 2007). The title ‘southern paralysis tick’ has been proposed for Ixodes cornuatus and ‘paralysis tick’ for Ixodes holocyclus, better reflecting their geographical distribution (Barker and Walker, 2014). The geographical distribution of Ixodes holocyclus is considered to be from east of Lakes Entrance in Victoria, along the east coast of Australia, up to the northern tip of the east coast to Cape York Peninsula extending variable distances inland but mostly less than 50 km from the coast (Fitzgerald, 1998). The high humidity requirement of the tick for development may explain the primarily coastal distribution. Sporadic cases of tick paralysis outside of known endemic areas have been described but these can largely be explained by a recent history of travel of the animal or owner (Whitfield et al., 2017). Exposure to recreational areas containing natural vegetation in an urban environment was a significant risk factor for dogs that developed tick paralysis in Brisbane (Gerasimova et al., 2018).
3.1 Isolation and characterisation of the salivary origin neurotoxin
The quest to understand the enigmatic molecular nature of the paralysing neurotoxin produced by Ixodes holocyclus has been long and tortuous but major knowledge gains have been made recently. A salivary-gland origin toxin was first hypothesised by Ross to be the paralysing agent (Ross, 1926). Ross correctly concluded this because: (i) he was unable to transfer the toxicity from a paralysed animal through injection of whole blood or body fluids into another dog or experimental animal (although the concentration of toxin in serum is probably extremely low) (Ross, 1926); (ii) injection of salivary gland extracts from engorged ticks from engorged ticks were shown to reproduce the disease (Ross, 1935). Multiple reports since the early work of Ross continued to demonstrate the toxin was produced in the salivary glands following tick engorgement, but the molecular nature of the toxin remained elusive (Kaire, 1966; Stone and Wright, 1981). The production of toxin in the salivary glands reaches peak levels after 3 to 5 days and this coincides with onset of progressive paralysis in the host (Goodrich and Murray, 1978; Murray and Koch, 1969). It was not until the application of modern chromatographic methods to the purification process that the causative neurotoxin was found to be a 5-6 kD protein molecule (Thurn et al., 1992). Thurn’s purification process concentrated the toxin such that it had a 313-fold greater biological activity than the crude tick extract starting material. Commercial TAS blocked binding of the 5-6 kD toxin to rat synaptosomes and bound to the toxin on western blots (Thurn et al., 1992). The authors maintained that the previous work (Stone et al., 1979; Stone and JH, 1987; Stone and Wright, 1979) on descriptions of a 40-80 kD causative toxin was probably compromised by the binding of the toxin to serum albumin (Thurn et al., 1992). The name holocyclotoxin (HT-1) was proposed to describe the first neurotoxin described from Ixodes holocyclus. A patent was granted for the HT-1 peptide sequence (SCTNPGKKRCNAKCSTHCDCKDGPTHNF GAGPVQCKKCTYQFKGEAYCKQ) and associated uses in 1997 (Broady et al., 1997).
3.2 Discovery of the holocyclotoxin peptides
Since the discovery of the HT-1 peptide as an important neurotoxin in the paralysis tick, over 20 closely related peptides that are also produced by Ixodes holocyclus have been described and their nucleotide sequences added to GenBank. These breakthroughs were made possible by next-generation sequencing technologies applied to the salivary gland transcriptome of the engorged paralysis tick, synthetic peptide chemistry and recombinant protein production techniques (Ong et al., 2016; Rodriguez-Valle et al., 2018). Not surprisingly much of this recent discovery work has taken place in Brisbane at the University of Queensland, Australia where tick paralysis is a significant regional problem.
The neurotoxicity of four synthetic holocyclotoxin peptides produced by automatic Fmoc chemistry and termed HT-1, HT-2, HT-3 and HT-4 was demonstrated in vivo in a neonatal mouse bioassay using 4-5 g mice (Rodriguez-Valle et al., 2018). HT-1, HT-2 and HT-3 were mildly toxic whilst HT-4 was highly toxic; subcutaneous injection of 30 µg of HT-4 induced severe and fatal respiratory paralysis within 2 hours. The mixture of HT-1, HT-2 and HT-3 was more toxic than when each peptide was injected individually, suggesting a possible synergistic mode of action (Rodriguez-Valle et al., 2018). Molecular aggregation of the holocyclotoxin peptides into homodimers and heterodimers was suggested by the authors as a reason for the previous reports of a higher molecular weight toxin, although no data was presented. The pre-synaptic neurotoxic activity of synthetic peptides (HT-1, HT-3 and HT-12) was demonstrated in vitro using isolated neuromuscular preparations from mice and was mediated by inhibition of neurotransmitter release via a calcium dependent mechanism, (Chand et al., 2016). However, extremely high concentrations of the synthetic peptides were required which made the authors suspect that these synthetic peptides were not folded entirely correctly or a different mechanism of action may apply (Chand et al., 2016).
Three-dimensional structural analysis of synthetic HT-1 peptide revealed a molecule with four disulphide bonds and a folded structure, although no in vivo toxicity data was reported by the authors for this synthetic peptide (Vink et al., 2014). HT-1 has structural similarity to scorpion neurotoxins and contains eight cross-linked cysteine residues giving it a ‘cysteine knot’ structure (Vink et al., 2014).
Successful recombinant expression of HT-1 in Saccharomyces yeast was reported by researchers at the University of Queensland (Karbanowicz et al., 2017). Although synthetic peptides with neurotoxic activity were produced by the same group, the recombinant methodology was considered a more cost-efficient production system to pursue in respect of commercial development of a vaccine. No in vivo toxicity data was reported for the recombinant HT-1. The authors presumed that due to the similar binding to rat synaptosomes in vitro of both the synthetic and recombinant versions that they would both have biological activity (Karbanowicz et al., 2017).
3.3 Mechanism of action of the neurotoxin
The physiological action of the neurotoxin produced by Ixodes holocyclus has been studied experimentally using isolated neuromuscular preparations. A presynaptic mode of action of the tick toxin was ascertained using electrophysiological studies using both synthetic peptides and native toxin performed on isolated mouse nerve-muscle tissue in organ baths (Chand et al., 2016). Tick toxin was demonstrated to affect entry of calcium into cells causing interruption of neurotransmission. However very high concentrations of individual synthetic peptides were required though and it was speculated that there may be synergistic action amongst the different holocyclotoxins (Chand et al., 2016).
The recent findings using synthetic toxin peptides are consistent with the previous studies of Cooper and Spence (1976) in mice and dogs using native toxin. Tick toxin was found to result in a temperature dependent reduction in neurotransmission at the neuromuscular junction, caused by an inhibition in the amount of acetylcholine released (Cooper and Spence, 1976). To demonstrate this effect, preparations of phrenic-nerve diaphragm muscle obtained from mice paralysed with ten Ixodes holocyclus nymph for 3.5 to 4.5 days were incubated in an electrolyte solution at various temperatures. The action of the tick toxin was studied by electrical stimulation of the isolated nerve preparations and measurement of the resulting twitch in the connected diaphragm muscle (Cooper and Spence, 1976). There was a delay of 6-7 hours between incubation of mouse nerve-muscle tissue in buffer containing the tick toxin extract and inhibition of the neuromuscular junction (Fitzgerald, 1998). The delay between exposure of the neuromuscular junction to tick toxin and paralytic effect is consistent with clinical signs typically observed in affected animals when treatment is begun and when toxin extracts are injected into experimental animals (Cooper et al., 1976). Cooper postulated that this delay could be due to either chemical modification of the toxin in vivo or simply a delay in reaching the site of action. Due to the temperature dependence and absence of binding of the neurotoxin at low temperatures, implementation of “controlled hypothermia” was advocated as a treatment of clinical paralysis. When considering clinical adoption of this recommendation Fitzgerald (1998) was careful to advise that to avoid compounding the generalised illness and depression associated with tick paralysis, core temperature should not be allowed to fall below 34-36C (Fitzgerald, 1998). In a literature review of the available knowledge on controlled hypothermia for treating tick paralysis it was concluded that until further research is conducted that paralysed animals not be exposed to excessively high or low temperatures (Fearnley, 2002).
Studies of isolated ulnar and tibial nerve preparations obtained from dogs with experimentally induced tick paralysis did not reveal any delay in nerve conduction velocities or pulse amplitudes in nerve trunks. Isolated muscle preparations from these dogs similarly did not reveal any change in action potential shape but a reduction in amplitude of the muscle contraction. This experimental information supported their hypothesis that the site of action of the tick toxin is in, or near, the neuromuscular junction (Cooper et al., 1976).
3.4 Anticoagulant
An anticoagulant found in extracts of engorged Ixodes holocyclus salivary glands has been described (Anastopoulos et al., 1991). The activity of the anticoagulant was found to be greatest when extracted from the gut of the tick, although limited activity was also found in salivary gland secretions. The high levels in the gut may be due to re-ingestion by the tick during feeding of the salivary gland origin anticoagulant (Anastopoulos et al., 1991). It is postulated that the anticoagulant assists in maintaining blood flow whilst the tick is feeding. The anticoagulant appears to have no clinical or pathological significance in animals.
3.5 Cardiac toxins
The presence of cardiac-acting toxins within the salivary secretions from Ixodes holocyclus was demonstrated in effects on heart function in live rats (Campbell et al., 2004). Prior incubation of tick toxin extracts with canine TAS prevented the cardiovascular effects in live rats and excised rat cardiac tissues (Campbell et al., 2004). A positive inotropic response was observed upon the in vitro cardiac tissues from rats and at higher doses arrhythmic responses in the right atria were also recorded in live rats. The responses of rat cardiac tissues to tick toxin were consistent with the mechanism of action being a blockade of potassium channels (Campbell et al., 2004). No histological or ultrastructural changes were identified in rats in which tick paralysis was experimentally induced by multiple tick attachment and engorgement. It is unclear whether the cardiac effects are due to different toxins to the holocyclotoxins that cause generalised paresis. Consistent with the peptide sequence homology (ie scorpion α-toxins), there is also some clinical similarity between symptoms of scorpion envenomation and Ixodes holocyclus with paralysis, cardiac dysfunction and pulmonary oedema features of both toxicities (Isbister and Bawaskar, 2014). A recent study examined cardiac biomarkers (NT-proBNP and cardiac troponin I) to further investigate if there is cardiac involvement in dogs with tick paralysis (Nicolson et al., 2016). The authors did not detect significant changes in circulating serum cardiac troponins in dogs with tick paralysis. The exact pathophysiology of the pulmonary oedema is yet to be clearly defined.
3.6 Toxic effects from injection of unfed ticks
There has been conjecture over the toxicity of unfed Ixodes holocyclus ticks when injected into mice. The toxicity of salivary gland extracts produced from unfed ticks was found in one study to be relatively high and declined during the first 24 hours after initiation of feeding only to resume toxicity at the end of feeding (Stone et al., 1979). However, work performed subsequently by a different group examining the toxicity of unfed ticks was in complete contrast to the work of Stone. No toxicity was observed from injection of unfed ticks into mice (Davey et al., 1988). Up to 20 unfed ticks were injected into each mouse and without toxicity. The absence of toxicity in mice injected with homogenates of unfed adult ticks was also demonstrated in two other studies (Goodrich and Murray, 1978; Kaire, 1966). Davey questioned how such a large difference could be observed between their studies and that of Stone who reported toxicity in unfed ticks.
3.7 Larvae toxicity
The newly hatched larvae can cause an intense skin irritation in humans and animals exposed to large numbers (Ross, 1935). Neurotoxicity resulting from the attachment of 500 larvae to 500 gram guinea pigs has been reported but it appears toxicity per larvae is much less than in adult ticks as large numbers of larvae are required (Oxer and Ricardo, 1942). Clinical neurotoxicity due to larvae infestation is possible but appears be a rare clinical event in domestic animals. One clinical case of tick paralysis was described in a 4.5kg domestic cat with an estimated 200-300 Ixodes holocyclus larvae attached (Fitzgerald, 2007). The clinical signs described were mild and the cat recovered within 48 hours following administration of canine TAS and removal of the larvae.
3.8 Nymph toxicity
Toxicity from attachment of nymphal stages of Ixodes holocyclus has been described in a dog (Ross, 1932) and experimental guinea pigs (Oxer and Ricardo, 1942). A six month old cocker spaniel was found to have ‘not less than 100’ engorging nymph attached around its eyes and face; the dog developed lethargy, mild hind limb paresis, but recovered without TAS and physical removal of all nymph (Ross, 1932). Five days later the same dog again had over 70 engorged nymph attached, exhibited mild hind limb paresis and once more spontaneously recovered after seven days (Ross, 1932). Guinea pigs weighing 400-500g in which 25 nymph were attached died of paralysis within 7 days whilst 1-12 nymphs was unable to cause paralysis (Oxer and Ricardo, 1942). Nymph may attach and engorge on dogs but scratching by the dog may dislodge many of the attached nymphs (Oxer and Ricardo, 1942). Nymphs have only minor significance in causing clinical paralysis in domestic animals although skin irritation may be more relevant.
3.9 Other
Extracts of the cuticle of unfed ticks were reported to be toxic (Doube et al., 1995). Toxicity was associated with the cuticle washings of fully engorged larvae, nymphs and adults. A respiratory paralysis was reported to occur in mice 9-10 hours after intraperitoneal injection (Jones, 1991). No further descriptions of this possible cuticle associated toxin have been published. Homogenates of eggs obtained from 17 different species of Ixodid ticks were lethal when injected into guinea pigs at a dose rate of approximately 300 mg/kg (Riek, 1957). Death was not associated with paralysis but appeared to due be a fatal generalised toxaemia, different to the paralysis syndrome seen with adult ticks. Egg toxicity is of no clinical relevance to domestic animals.
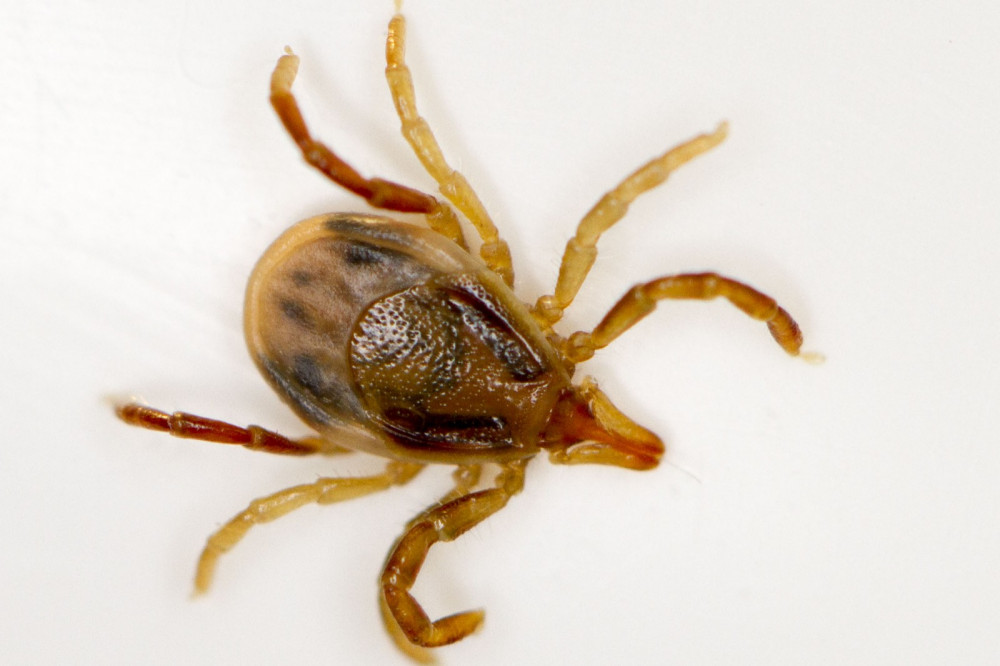
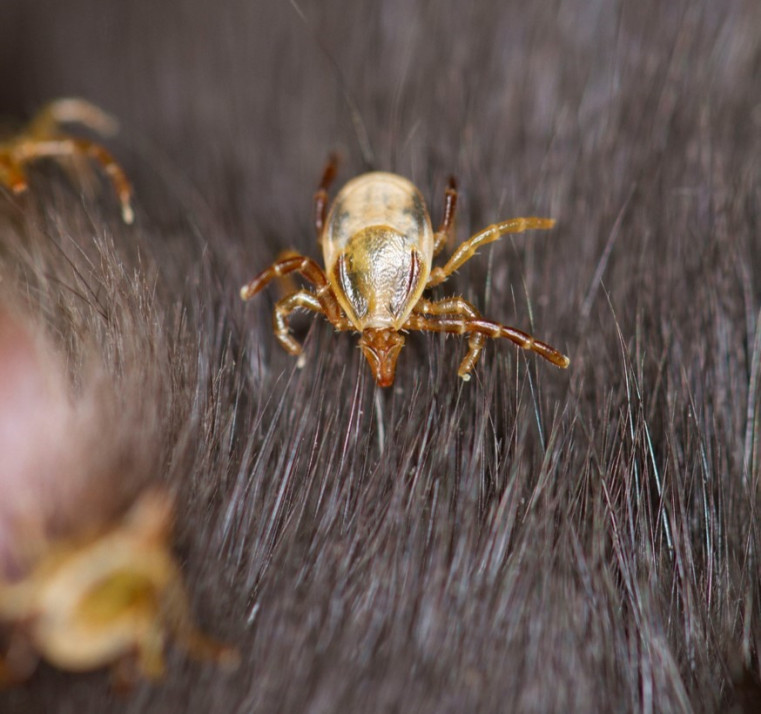
4.1 Dogs
Dogs are perhaps the most commonly affected of the companion animals and the clinical signs have been well described both in experimental and naturally occurring cases. A progressive and generalised lower motor neuron paralysis is one of the predominant clinical features in dogs, advanced stages can lead to respiratory muscle failure. The toxin can also have a profound effect on the gastrointestinal system, resulting in megaoesophagus and small intestinal ileus. Regurgitation is a key clinical feature however in the early stages in dogs and vomiting can be the only presenting clinical sign. Local neurological effects are also described. The extent of lower motor neurone paralysis and respiratory signs can be conveniently scored (Tables 2 & 3) for clinical staging purposes.
Experimental studies where paralysis ticks were attached to dogs have provided very detailed clinical descriptions of the typical sequence of events. In such a study designed to document the clinical signs of tick paralysis in eight Beagle dogs, each with 3-4 ticks attached, the interval from attachment to the first clinical signs was 5.5 to 7.0 days (Ilkiw et al., 1987). Seven of eight dogs developed clinical disease consisting of ascending flaccid motor paralysis and died within 18-32 hours of onset of signs (Ilkiw et al., 1987). One dog with three ticks attached did not die nor show any clinical signs of paralysis which the authors considered was due either to immunity of lack of toxin production by the ticks. The authors noted a tendency for dogs which developed clinical signs early to more rapidly progress to fatal paralysis (Ilkiw et al., 1987). The first noticeable clinical signs were for those dogs that barked, a mild hoarse or husky tone to the bark, which was then followed by hind limb ataxia. The dogs appeared healthy, ate and drank normally, and did not vomit. As the paresis progressed to also involve the front legs, 20% of dogs were observed to vomit and retching noted in some dogs if food was placed in front of them (Ilkiw et al., 1987). As paralysis progressed the dogs were unable to right themselves and adopted lateral recumbency, respiration was associated with grunting noises and swallowing reflexes were affected (Ilkiw et al., 1987). In the terminal stages the mucous membrane colour was grey, pupils dilated and death occurred within two hours of loss all withdrawal reflexes (Ilkiw et al., 1987).
Focal neurological defects associated with or without generalised paralysis have been described in animals with tick paralysis (Holland, 2008). Unilateral facial paralysis and anisocoria were described in 27 clinical cases occurring in dogs and cats (Holland, 2008). When facial paralysis and anisocoria was present the tick attachment site was always located on the head or neck, and always ipsilateral to the side of facial paralysis. The facial nerve paralysis may result in a reduced or absent palpebral reflex and secondary exposure keratitis or corneal ulceration can occur. Asymmetrical focal neurological deficits were a consistent finding in a 9.4% (27/286) of clinical cases in dogs and cats presented with naturally occurring tick paralysis (Holland, 2008). The focal neurological lesions were described to persist for many days and sometimes weeks after resolution of generalised signs of tick paralysis (Holland, 2008).
Canine mortality associated with tick paralysis cases treated by general practice veterinarians has been reported as 5% (Atwell et al., 2001). Overall canine mortality in cases treated at emergency and specialist clinics has yet to be reported.
4.2 Cats
Cats are frequently affected with tick paralysis and although the syndrome is similar to dogs there are subtle clinical differences (Schull et al., 2007). In dogs, lower motor neurone signs and gait changes predominate in the early stages, whilst in cats respiratory signs tend to be more prominent (Leister et al., 2018). Early clinical signs in cats, prior to generalised paralysis, such as dysphonia or aphonia due to laryngeal paralysis have been observed in cats (Schull et al., 2007). Hind limb paresis and ataxia are also common presenting signs which may progress to recumbency and generalised paralysis (Schull et al., 2007). Curiously the cat’s tail may remain unaffected in many cases. Respiratory signs in cats may present very differently, either as laryngeal dysfunction causing a function upper airway obstruction, or respiratory muscle failure which may lead to hypoventilation requiring mechanical ventilation. Cats often appear distressed and anxious presumably due to functional upper airway obstruction. Advanced gait score cats have a higher risk of mortality due to respiratory muscle failure, gait score 3 and 4 cats should be monitored closely for unsustainable effort or hypoventilation (Leister et al., 2018). Cats may also present with a combination of respiratory signs. Stress can further exacerbate an acute upper away obstruction requiring immediate general anaesthesia or heavy sedation and endotracheal intubation. If this is not implemented quickly acute decompensation and cardiopulmonary arrest may ensue (Schull et al., 2007). In contrast to dogs, megaoesophagus has not been described in cats (Day and Schull, 2008). Gastrointestinal signs are infrequently described, and although paralysed cats are at risk of aspiration pneumonia this is rarely observed in cats.
In a retrospective study of 2077 cases of tick paralysis in cats presented to a specialist veterinary practice in Brisbane the overall mortality was 2% (Leister et al., 2018). In cases where the recommended treatment was declined by the owner the overall mortality was 3%. In general, cats presenting with tick paralysis have good prospects for complete recover following specific treatment (Leister et al., 2018).
The diagnosis of tick paralysis is based on the presence of typical clinical signs, and evidence of a tick or tick attachment crater. A retrospective examination of 325 cases of tick paralysis in dogs and cats presented to six veterinary practices in Sydney, NSW, revealed that 10% of dogs and 4% of cats presented with no tick visible; 77% of dogs and 81% of cats had one tick; 8% of dogs and 15% of cats had two or more ticks; whilst 4% of dogs had more than three ticks (Westwood et al., 2013). More than 70% of ticks attach to the head and neck region in experimentally and naturally infested dogs, whilst only 10% of ticks attached to the ventral body (Atwell et al., 2000). Dogs with long hair coats were found to be more likely to have more ticks attached than shorter hair coat dogs (Atwell et al., 2001). The tick location data in cats found that 90% were found from the shoulder forward, with the highest percentage found around the neck (48%), followed by the head (25%). In the group of cats who had coat clipping performed 9% had a second tick located and this group of cats also had a reduced risk of mortality compared to the cats who didn’t have coat clipping performed. The Australian Paralysis Tick Advisory Panel has categorised the presentation of tick paralysis cases into two broad categories.
5.1 Clinical signs of tick paralysis with or without evidence of a tick or tick crater
Diagnosis of tick paralysis in geographical areas where Ixodes holocyclus is endemic and seasonal, usually pose little difficulty. In many cases the presence of an engorged tick or a tick attachment crater and typical clinical signs are diagnostic. The easily recognisable signs of ascending flaccid lower motor neurone paralysis often accompanied or preceded by dysphonia and/or vomiting and/or regurgitation and/or gagging and retching are arguably pathognomonic (Westwood et al., 2013). A typical history involves residence in a tick habitat or a visit to in the previous three to six days. The speed of onset of these signs and the rate of progression appears variable.
The diagnosis of tick paralysis can be extremely challenging when a paralysis tick or tick crater cannot be located. Particularly for those cases occurring outside of a known paralysis tick area (Kelers et al., 2012) or those with limited or unusual signs. Locating an engorged Ixodes holocyclus (or cornuatus) will support the diagnosis but when a tick is not found differential diagnoses such as myasthenia gravis, acute polyradiculoneuritis, snakebite (especially brown snake, Pseudonaja textilis), tetrodotoxin, spinal pathology and botulism may need to be considered.
Presentation of dogs to veterinarian with suspicious clinical signs of tick paralysis such as gastrointestinal signs, respiratory changes or lower motor neurone paralysis as individual presenting signs or in combination and recent exposure to a geographical tick region - but in which no tick can be found - are very challenging for veterinarians to manage and clients to understand. Repeated searching of dogs is essential and total body clipping of all hair may facilitate the location of engorged ticks (Fitzgerald, 1998).
5.2 No clinical signs of tick paralysis with evidence of a tick or tick crater
The presence of an engorging paralysis tick on a dog or cat may not be associated with clinical signs. Prompt removal of the offending tick is indicated. However, tick removal may still lead to the development of paralytic signs within the next 1-2 days. The Australian Paralysis Tick Advisory Panel recommends that veterinarians adopt a risk-benefit approach to these cases. Consideration should be given to the history, signalment, likelihood of disease progression, access to veterinary attention and possibility of adverse systemic reactions to TAS.
5.3 Other
Dogs with tick paralysis may present with an atypical history of vomiting or regurgitation and no apparent neuromuscular weakness (Campbell and Atwell, 2001) (Malik et al., 1988). Careful veterinary diagnostic evaluation to exclude other causes of vomiting is essential along with coat clipping combined with regular and repeated whole-body searches for ticks. Evidence of megaoesophagus (Figures 7, 8), defined as oesophageal dilation on radiographs, was found in 70% (28/40) of dogs diagnosed with tick paralysis (Campbell and Atwell, 2001). In another study utilising only a single left lateral chest xray at admission, 19% of dogs had radiographic evidence of megaoesophagus (Day and Schull, 2008). The development of megaoesophagus was not significantly associated with the tick location being on the neck or throat region. Megaoesophagus was more likely to be detected in older dogs with tick paralysis and recovery of oesophageal function lagged behind that of generalised tick paralysis (Campbell and Atwell, 2001). Vomiting and regurgitation without classical signs of paralysis has been described and a series of three such case presentations has been described (Malik et al., 1988). The extreme difficulty in obtaining a diagnosis was highlighted in this report. Multiple days hospitalisation elapsed before diagnosis of tick paralysis, death of two of the dogs, and exploratory laparotomy surgery performed in one case in an attempt to diagnose the cause of the gastrointestinal upset which later died (Malik et al., 1988). None of the three dogs were reported to have displayed signs of generalised paralysis demonstrating the difficulty veterinarians face with atypical tick paralysis cases.
The frequency of megaoesophagus in dogs with tick paralysis and the radiographic visibility on plain views of this condition would suggest that its identification may be a useful diagnostic screening test in suspect cases. Thoracic radiographs could be used to support administration of TAS in cases where no tick can be found.
There is potential need to develop a specific immunological diagnostic test for the presence of the holocyclotoxin peptides in serum or urine of paralysed animals. An immunoassay to detect toxin antigen – similar to those used for snake venom assays – may assist in better understanding the toxin, support a diagnosis when no tick can be found and help to rationalise TAS therapy (Padula and Winkel, 2016).
6.1 Seasonality
Tick paralysis is most commonly reported in the spring and summer, but cases may occur all year round in the warmer endemic regions. In a study of 2077 cases in cats in south east Queensland the highest number was seen in spring (66%), followed by winter (23%), with summer (9%) and autumn (2%) having fewer cases (Leister et al., 2018). In another study around Sydney, season was also important with 92% of tick paralysis cases in both dogs and cats presented for treatment in the spring and summer (Westwood et al., 2013). The most common presentation in dogs (46%) and cats (47%) was for a mild gait abnormality. The median hospitalisation time for dogs and cats was two days. The authors also reported that 9% of dogs and 11% of cats had been treated on at least one previous occasion for tick paralysis (Westwood et al., 2013). Based on these reports it is likely that regional geographical climate variation affects the seasonality of tick paralysis. Cases of tick paralysis do occur outside of the typical season and veterinarians need to be vigilant and maintain a high suspicion index to ensure diagnosis, prompt and specific treatment (Fitzgerald, 1998).
6.2 Geographical region
Cases of tick paralysis may occur outside of a known endemic geographical areas for various reasons (Beveridge, 1991). These may be caused by previous exposure to a tick infested area, transportation of tick infested materials with ensuring fomite transmission, or changing geographical distribution of the causal ticks. Two separate cases of tick paralysis caused by Ixodes holocyclus in dogs that had never left the Melbourne area were described as occurring in the outer east of Melbourne, which is not a known paralysis tick area (Beveridge, 1991). Straw bedding material originating from Queensland was suspected to have carried questing Ixodes holocyclus ticks which subsequently caused tick paralysis in a dog in Melbourne (Beveridge, 1991). A dog was imported into New Zealand from Sydney, Australia that developed clinical signs of tick paralysis and died within days of arriving in New Zealand; presumably the dog was transported with a tick already attached (Hutton, 1974).
6.3 Australian Tick Paralysis Advisory Panel
An expert consensus group called the Australian Tick Paralysis Advisory Panel has recently published an agreed set of recommendations for the diagnosis, treatment, management and prevention of tick paralysis in animals (Anon, 2016). The resulting evidence-based guidelines consist of information sourced from peer-reviewed publications, reported experiences, and expert opinions. In the absence of strong evidence, statements have been made based on the clinical expertise of the Panel.
7.1 Tick antiserum
The specific veterinary treatment for cases of tick paralysis has been tick removal and administration of canine TAS harvested from hyperimmune donor dogs. Despite its widespread use there is only very limited published scientific data to support its effectiveness. Almost all clinical case studies have not included untreated tick infected control animals. However there is strong anecdotal belief amongst veterinarians that treat many cases of tick paralysis that the TAS is effective. Early administration of TAS is advocated to obtain the best patient survival and minimise complications (Fitzgerald, 1998). Ross first described the use of canine TAS for the treatment of tick paralysis in dogs and commented that over 75% of cases survived with TAS, but no detailed case data was published (Ross, 1935).
Canine TAS has historically been prepared based on the original methods developed by Ross (Oxer, 1948). Large breed dogs are progressively exposed to increasing numbers of ticks until they develop sufficient immunity to withstand engorgement of 30 or more ticks simultaneously (Oxer, 1948). Serum (or plasma) is then harvested from the dogs at regular intervals following boosting of immunity by applying additional ticks to each dog. Historically tests of serum neutralising potency were performed using a death-as-endpoint adult mouse protection assay (Kaire, 1965; Oxer, 1948; Ross, 1927b, 1935) and reported as protective potencies, typically of 3-50 mouse LD50 per mL of serum. A neonatal mouse protection assay that used a 24 hour paralysis index was later described and adopted for commercial serum batch release testing (Stone et al., 1982). Neonatal mice differ in their response to tick extracts compared to adult mice, with paralytic signs being more readily observed; adult mice tend to die quickly with minimal paralytic signs (Stone et al., 1982). More recently an indirect enzyme immunoassay has been used to determine specific IgG antibody levels (Hall-Mendelin et al., 2011b) to tick proteins and this has been adopted by regulatory authorities as a potency test (Westwood et al., 2013).
Only one published study could be found that compared outcomes of untreated control dogs with experimentally induced tick paralysis, and dogs treated with TAS (Ilkiw and Turner, 1988). Six non-engorged Ixodes holocyclus ticks were applied to each of 40 dogs in a trial; 20 dogs subsequently developed signs of paralysis, and four dogs were then allocated to one of five different treatment groups including an untreated control. It was not stated if the ticks were removed from the dogs prior to applying the treatment and the stage of paralysis is not clear. The results revealed that dogs that received no tick TAS all (4/4) died within 3.3 hours. Dogs that received TAS only and no other drugs 75% (3/4) died within 38.3 hours. TAS prolonged the time to death. The surviving dog took 57.8 hours to walk again and a further 4 days to be fully recovered. Four dogs were treated with TAS plus dexamethasone (0.5 mg/kg) every 12 h 75% (3/4) of the dogs that survived. Treatment with TAS plus promethazine resulted in 50% (2/4) of dogs surviving. Whilst treatment with TAS plus phenoxybenzamine (1 mg/kg) resulted in 100% (4/4) dogs surviving. The overall results of this study are difficult to interpret as the numbers are small, some details are missing and only half of the dogs that had ticks applied became affected with tick paralysis. The authors reported that based on the phenoxybenzamine results veterinary practitioners had since adopted that as a treatment alongside TAS (Ilkiw, 1983). Despite the encouraging preliminary clinical report on the use of phenoxybenzamine it appears to have not been widely accepted and its use is not included in more recent treatment recommendation reviews (Fitzgerald, 1998; Jones, 1991)
The time from administration of TAS to clinical improvement has been reported as a minimum of 12 hours (Fitzgerald, 1998; Ilkiw and Turner, 1988). A worsening of clinical signs during this time period commonly occurs until improvement is noted.
All commercial TAS products in Australia contain either phenol or cresol as a preservative to limit microbial growth. Administration of large volumes of TAS to low bodyweight animals may lead to high serum levels of phenol, which may create a theoretical risk of phenol toxicity, although this has not yet been described.
The dose of TAS administered to animals with tick paralysis has long been a subject of discussion by veterinarians. However consideration must be given to the variable potency of canine TAS, which is a recognised issue (Westwood et al., 2013). Veterinarians typically adopt one of two regimes for dosing with TAS. Some have advocated a dose proportionate to bodyweight in the range of 0.5 – 1.0 mL/kg; others have recommended a fixed minimum volume of 10-20 mL per animal or per tick (Fitzgerald, 1998; Webster, 2014a). Treatment with a single large dose of TAS as early as possible in the disease process is recommended rather than attempting to titrate the dose to clinical effect (Fitzgerald, 1998). Intravenous administration is essential due to the slow absorption of large molecular weight serum immunoglobulin proteins from subcutaneous or intramuscular sites. Intraperitoneal administration of canine TAS is used by some veterinarians to minimise the risk of anaphylactic reactions to the canine proteins, particularly in cats (Schull et al., 2007) however controlled trials are required to thoroughly evaluate this hypothesis.
7.2 Tick antiserum reactions
Canine TAS is a biological substance that is not innocuous when administered to either cats or dogs. The administration of canine TAS is reported to have occasional acute adverse effects in dogs, but these events appear more likely to occur in cats and of greater clinical severity (Schull, 2007). Controlled studies into the mechanisms underlying TAS reactions in cats and dogs are sparse and much has been speculated about the pathogenesis. Factors such as rate of administration, total volume administered, recipient species (dog, cat), method of TAS preparation (clotted blood, recalcified plasma, fractionated serum), and methods for detecting reactions almost certainly influence the described signs.
The Australian Paralysis Tick Advisory Panel has defined two types of acute reactions to TAS administration: (i) bradycardia, pale mucous membranes, hypotension, weakness, depression and reduced heart sounds; or, (ii) tachycardia, injected mucous membranes, anxiety, piloerection, swelling of the lips, cutaneous wheals, erythema, vomiting, diarrhoea, coughing and dyspnoea (anaphylactic/anaphylactoid reaction). Reactions of the first type are presumed to be anaphylactic or anaphylactoid in nature and primarily occur in cats, whilst of the second type are appear to cardiovascular in origin and are observed mostly in dogs.
7.2.1 Infusion rate in dogs
The rate of infusion of TAS has been shown to influence the extent of acute adverse reactions in dogs. Under controlled experimental conditions, Schull (2008) observed acute TAS reactions in 21/24 (80%) of dogs administered TAS over 5-minutes, but did not detect changes in any (0/8) control dogs given fresh frozen plasma (FFP) under the same conditions. Heart rate was significantly lower in dogs whilst receiving TAS than FFP, whilst respiratory rate was significantly increased in dogs receiving TAS than for FFP (Schull, 2008). In a second study assessing the effect of TAS Schull (2008) found that dogs given TAS over 5-minutes had significantly more adverse effects than if TAS was given over 20 minutes (Schull, 2008). Systemic arterial blood pressure measured using Doppler technology declined to 48% pre-infusion when TAS was given over 5-minutes compared to 24% (P=0.011) for the 20-minute infusion groups (Schull, 2008). The Australian Paralysis Tick Advisory Panel (Anon, 2016) recommendation is to administer canine TAS as a dilute solution in sterile saline over 20 minutes.
7.2.2 Antiserum preparation method
Differences in the methods used to prepare TAS likely contribute to the incidence of adverse reactions. Serum produced from centrifuged clotted whole blood is not identical in its constituents to serum produced by recalcification of citrated (or oxalated) platelet poor plasma. Studies conducted in normal dogs have revealed transient acute adverse reactions to both TAS and normal serum (Schull, 2008). Schull observed acute reactions in 80% (4/5) of dogs receiving 1 mL/kg of canine TAS and also in 80% (4/5) of dogs receiving 1 mL/kg of normal canine serum (derived from clotted whole blood) intravenously. Reactions however were not observed in dogs receiving either 1 mL/kg of normal canine plasma (0/5) or normal saline (0/4) (Schull, 2008). The effect of the preservative phenol was also studied under the same experimental conditions by Schull who administered 0.25% phenol saline and 0.25% phenol plasma; however no adverse effects occurred, indicating phenol is unlikely to be the cause (Schull, 2008).
The nature of adverse reactions in dogs to canine TAS intriguingly parallels acute reactions described in humans administered serum from clotted blood. Studies were conducted in Australia during the 1940s to better understand why there was a higher incidence of adverse reactions in humans following intravenous administration of serum from clotted blood compared to plasma (Reid and Bick, 1942). Work conducted at Walter & Eliza Hall Research Institute demonstrated that serum, produced by the clotting of whole blood and centrifugation, resulted in production of vasoactive substances. In contrast, no vasoactive effects were observed in vitro on vascular tissue preparations when serum was prepared by recalcification of citrated plasma. The vasoactive (vasoconstrictive) substances were determined to be produced by platelets during the clotting process. The findings of Reid and Bick (1942) were pivotal in the development of a dried human serum product (derived from centrifuged plasma) as the exclusive blood substitute product used for Australia’s frontline medical second world war effort (Cortiula, 1999). The adverse phenomena observed in humans to serum produced from clotted blood had been known since the 1900s as the Brodie-phenomena (Brodie, 1900).
Serotonin may be the principal vasoactive substance causing adverse reactions following TAS treatment in dogs. Serotonin is released from platelets during clotting of whole blood and concentrations in serum are at 20 to 100-fold higher than in platelet free plasma (Jones and Kalman, 1980) . The volume and rate of administration of TAS likely determines the resulting pharmacological actions due to the rapid onset and short duration of action of serotonin (1-2 minutes) (Jones and Kalman, 1980). The measurement of serotonin concentrations in TAS and canine serum products could provide further evidence as to its role in acute TAS reactions. Experimental administration of serotonin receptor antagonists prior to TAS may also provide evidence to the importance of serotonin in TAS reactions.
The data of Schull is further evidence that serum itself may be the culprit for the acute cardiovascular adverse reactions in dogs. It is noteworthy that the original description of the method for TAS production in dogs used recalcification of oxalated plasma which may have inadvertently minimised reactions (Oxer, 1948).
7.2.3 Reactions in cats
Cats that receive canine TAS are undergoing a xenotransfusion and this poses inherent immunological challenges to the cat both in the short and long term. The effect of administration of canine TAS on mortality in 2077 cats diagnosed with tick paralysis was recently described (Leister et al., 2018). An overall mortality of 8% was observed in cats that did not receive TAS, compared with 2% in the group who did receive TAS combined with the recommended veterinary treatment. The overall incidence of adverse reactions in cats to canine TAS was 9%, varying from mild transient changes to sudden cardiopulmonary arrest. The mortality rate in cats that had a reaction to TAS was 6%, compared to a morality rate of 1% if no reaction to TAS was documented (Leister et al., 2018). In another study, the acute death of five cats following intravenous injection of canine TAS was reported by a veterinary practitioner, despite pre-treatment of each cat with antihistamine, steroids and adrenaline (Fitzgerald, 1998). It can be concluded that canine TAS has potentially lethal effects when administered intravenously to cats.
7.2.4 Preventing reactions
When TAS was administered (1 mL/kg) as a five-minute infusion to normal dogs, premedication with either atropine (0.1 mg/kg, i.v.) or adrenalin (0.01 mg/kg, s.c.) failed to prevent a decrease in mean systolic arterial blood pressure to below the normal reference range for dogs (Schull, 2008). When TAS was administered as a 20-minute infusion, mean systolic arterial blood pressure remained within the normal reference range, regardless of premedication given. Schull (2008) observed that significantly fewer dogs exhibited acute side effects following the 20-minute (25%; 3/12) compared to the five-minute (83%; 10/12) TAS infusion timeframe. Schull concluded that adverse effects were more likely related to the rate of infusion of TAS and were not preventable by atropine or adrenalin (Schull, 2008). Further work by Schull on the cardiovascular effects of commonly used pre-medications (adrenaline, atropine, acepromazine) revealed that they can all have deleterious cardiovascular effects, with no benefit of prevention of TAS reactions. Consequently, the use of adrenaline and/or atropine as premedication is no longer recommended. Although warming of TAS to body temperature prior to administration is also recommended to minimise reactions (Anon, 2016).
The physiology of TAS reactions in dogs has previously been suspected to result from triggering the Bezold-Jarisch reflex via activation of intra-cardiac receptors (Zucker and Cornish, 1981). In contradiction to this, the pathophysiology of TAS reactions has since been shown to be inconsistent with the Bezold-Jarisch reflex as atropine administration was not preventative when given in controlled physiological studies (Schull, 2008).
7.3 Supportive care including acaricidal treatment
General supportive veterinary care of tick paralysis cases is recognised as an important part of treatment. Patient survival has been improved from 75% as reported in the early years (Ross, 1935) of tick paralysis treatment where TAS was heavily relied upon, to the modern era where 95% survival is expected (Atwell et al., 2001). Survival in cats has shown to be even higher than dogs with a reported survival of greater than 97% (Leister et al., 2018). Improvements in hospitalisation and supportive therapies (eg mechanical ventilation) have likely led to these better outcomes. The generalised paralysis caused by toxins produced by Ixodes holocyclus results in a range of pathophysiological disturbances to multiple body systems throughout the body, and these require considered management to optimise patient outcomes.
Location, removal and identification of paralysis tick(s) are important and necessary preliminary treatment procedures. Removal of the tick(s) should be done as soon as possible to limit further absorption of toxin by the patient. Clipping all the hair off animals is recommended to located ticks. The most common site for tick attachment is around the head and neck but occasionally ticks are reported as located inside the roof of the mouth or even inside the anal sphincter (Jones, 1991). A recent report in 2077 cats with tick paralysis found at 90% were locatewd from the shoulder forward. Ideally, identification of the tick should be performed to ensure that it is Ixodes holocyclus or cornuatus, which are the only two species known to cause tick paralysis; use of a dissecting microscope and anatomical key are essential for accurate identification (Barker and Walker, 2014). Many other non-toxic Ixodes sp and non-Ixodes ticks may also be found on dogs and cats in throughout Australia which can create diagnostic confusion.
Oral isoxazoline acaricidal preparations (Table 4) appear effective in killing already attached engorging ticks, but the process may take up to 24 to 72 h, and does not necessarily guarantee that paralysis will not develop in non-immune dogs (Fisara and Webster, 2015). Physical removal of engorged ticks from animals not yet showing clinical signs frequently leads to signs of paralysis within 1-2 days. Topical fluralaner (Bravecto® Spot-On for dogs and cats) is now on the Australian market and has been shown to kill any paralysis ticks in situ within 72 h and application to all suspect or diagnosed cases may be beneficial (Fisara et al., 2018; Kilp et al., 2016).
The use of acaricidal washes, dips or application of topical insecticides to diagnosed or suspect cases of tick paralysis in dogs has previously been advocated (Jones, 1991). Complete immersion of a sedated animal in synthetic pyrethroid and organophosphate solutions typically used for cattle has been adopted by some veterinarians, with or without prior clipping of the hair coat (Jones, 1991). This practice may be of benefit in cases where no tick can be found, and the clinical signs and suspicion index are consistent with tick paralysis. However, there is no published data to support the efficacy of this acaricidal treatment in preventing the onset of tick paralysis.
Intravenous fluid therapy using a balanced electrolyte such as Hartmann’s or Plasmalyte 148 solutions administered at maintenance rates is indicated for patients that are unlikely to consume oral fluids within the following 24 hours (Webster, 2014b). Fluid therapy must be monitored to avoid fluid overload which may exasperate or lead to development of clinical pulmonary oedema (Webster, 2014b).
Bladder paralysis and the inability to pass urine may require management through either manual expression or catheterisation. Facial paralysis may lead to dry eyes and ulceration; the use of ocular lubricants may be necessary. Mild sedation may be useful to reduce anxiety and facilitate clipping and radiography; acepromazine (0.05 mg/kg) has been reported as adequate and useful (Jones, 1991).
Respiratory support may be required in some patients depending upon case severity, including intranasal or tracheal oxygen and/or mechanical ventilation (Webster et al., 2013c). The use of mechanical ventilation was shown to improve survival rates but is costly and labour intensive to manage. In a study of 54 dogs and 7 cats that were ventilated due to tick paralysis the mean duration of ventilation was 23 hours, but ranged from 3 to 144 hours (Webster et al., 2013a; Webster et al., 2013c). When cases euthanised on cost basis were excluded, 75% of ventilated animals survived (Webster et al., 2013c). Improved survival was noted for animals with hypoventilation but appeared less effective for animals when hypoxaemia was present (Trigg et al., 2014) with only 50% survival with hypoxaemia and 90% for hypoventilation (Webster et al., 2013c). Cats who require mechanical ventilation for tick paralysis have a reported survival rate of 82%, yet if mechanical ventilation was recommended but not implemented the survival was only 12% (Leister et al., 2018). These cases included cats with hypoventilation, severe TAS reactions and following cardiopulmonary arrest.
Dogs with tick paralysis have been shown to have varying degrees of left sided heart failure (Campbell and Atwell, 2003). Dogs with tick paralysis had normal systolic blood pressure, radiographic evidence of pulmonary venous congestion and peri-bronchial fluid infiltration, and a reduction in echocardiographic derived functional indices (Campbell and Atwell, 2003). Despite the recognition that heart failure may be occurring no specific cardiac treatments or medications are currently recommended (Anon, 2016).
Antibiotics would appear to be useful for management of existing aspiration pneumonia in paralysed animals. Evidence for this was found in the post-mortem examination of 25 dogs that had severe respiratory failure in which all dogs had histological lung changes and 15/25 had bronchopneumonia (Webster et al., 2013b).
Pulmonary oedema and congestion were noted in 36% (9/25) dogs euthanised for tick paralysis and this probably contributes to the development the hypoxaemia observed in some patients (Webster et al., 2013b)(Figure 5,6). Pulmonary oedema is a consistent finding in animals affected with tick paralysis. Left lateral chest xrays of 75 dogs presenting with tick toxicity revealed that 38% had signs of pulmonary congestion manifest as pulmonary vein dilation placing them at risk of developing pulmonary oedema (Day and Schull, 2008). It is unclear what the pathophysiology of this process is but it is an ongoing area of research interest (Webster, 2014a). Treatment of pulmonary oedema with diuretics may appear logical but diuretics raise the potential for other fluid therapy complications and has not be recommended for management of pulmonary oedema from tick paralysis (Webster, 2014b). The investigation of cardiac biomarkers in dogs with tick paralysis provides further evidence that the pulmonary oedema is non-cardiac in origin (Nicolson et al., 2016).
Oesophageal dysfunction and megaoesophagus appear to be relatively common in dogs with tick paralysis resulting in vomiting and regurgitation (Campbell and Atwell, 2001; Malik et al., 1988). Secondary oesophagitis may develop with megaoesophagus and medications such as cimetidine or omeprazole to reduce acidity of gastric secretions may be indicated (Campbell and Atwell, 2001). When introducing oral fluids and food during recovery feeding from an elevated position is thought to provide gravity assisted oesophageal movement. Anti-vomiting medications such as metoclopramide and maropitant are often given to tick affected animals but their efficacy for preventing aspiration pneumonia remains unproven (Webster, 2014b). It is recommended that tick paralysis affected animals should not be fed fluids or solids by mouth until such time that neuromuscular function has been sufficiently restored that aspiration pneumonia will be avoided (Webster, 2014b).
Despite some animals showing apparent recovery from generalised tick paralysis, occasional cases of unexplained sudden death have been reported to occur within a few weeks of recovery. Stressful stimuli, however mild, have been reported to precipitate sudden death (Fitzgerald, 1998; Ilkiw, 1983). Some of these deaths may be explained by undiagnosed bronchopneumonia and peracute respiratory distress. A convalescent period of two weeks post-treatment with only non-stressful activity has been recommended to minimise sudden unexpected deaths (Ilkiw, 1983). One speculated mechanisms for triggering sudden death may be cardiac arrythmia. Electrocardiographic abnormalities have been recorded in dogs recovering from tick paralysis (Campbell and Atwell, 2002). A prolonged QT interval was observed in dogs at the time of presentation for tick paralysis, at 24h post treatment, and at discharge from veterinary care when each animals results were compared to QT interval measured 12 months after recovery (Campbell and Atwell, 2002). T wave morphology was also altered in dogs at the time of presentation. Resolution of ECG changes lagged behind that of apparent clinical recovery. The authors concluded that prolongation of the QT interval seen on ECG likely predisposes dogs to sudden death from ventricular tachycardia, similar to long QT syndrome diagnosed in humans (Campbell and Atwell, 2002). A similar prolongation of the QT interval was also measured in the spectacled flying fox, a native bat species which is commonly affected with tick paralysis (Campbell et al., 2003). Dogs with non-severe clinical tick paralysis may still be predisposed to sudden death because of the delayed recovery from ECG abnormalities, and that TAS does not appear to reverse this ECG pathology (Campbell and Atwell, 2002). The incidence of sudden unexplained death in dogs recovering from tick paralysis is not known but a prospective survey of 500 cases of tick paralysis in dogs found nearly half of the 33 cases that ultimately died were reported by veterinarians as having been ‘unexpected’ (Atwell et al., 2001). Further studies are needed to better understand the nature of the cardiac events that may lead to sudden death in treated animals.
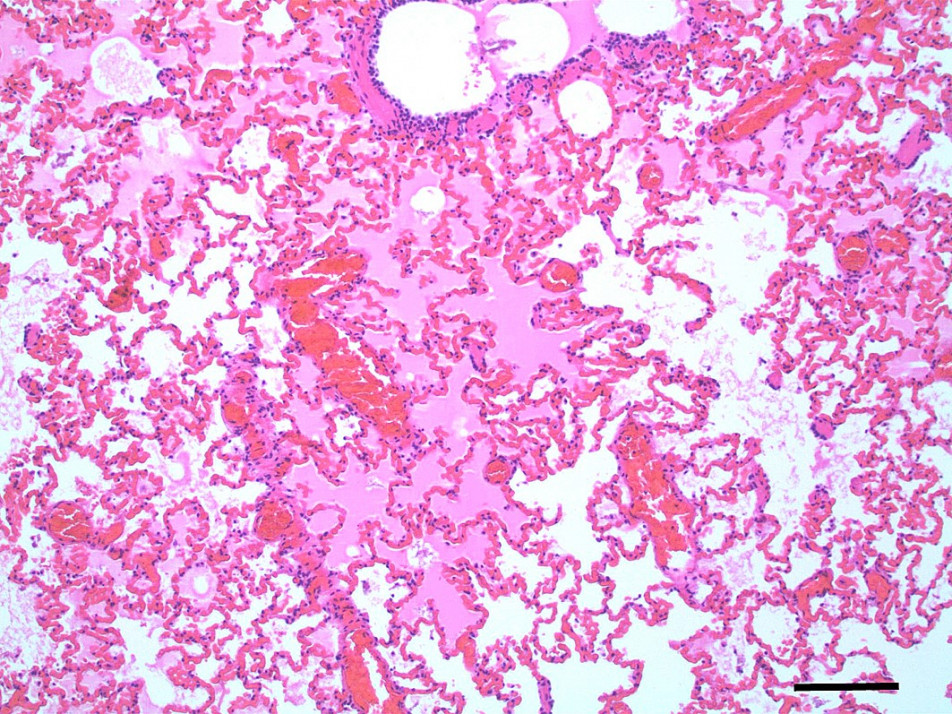
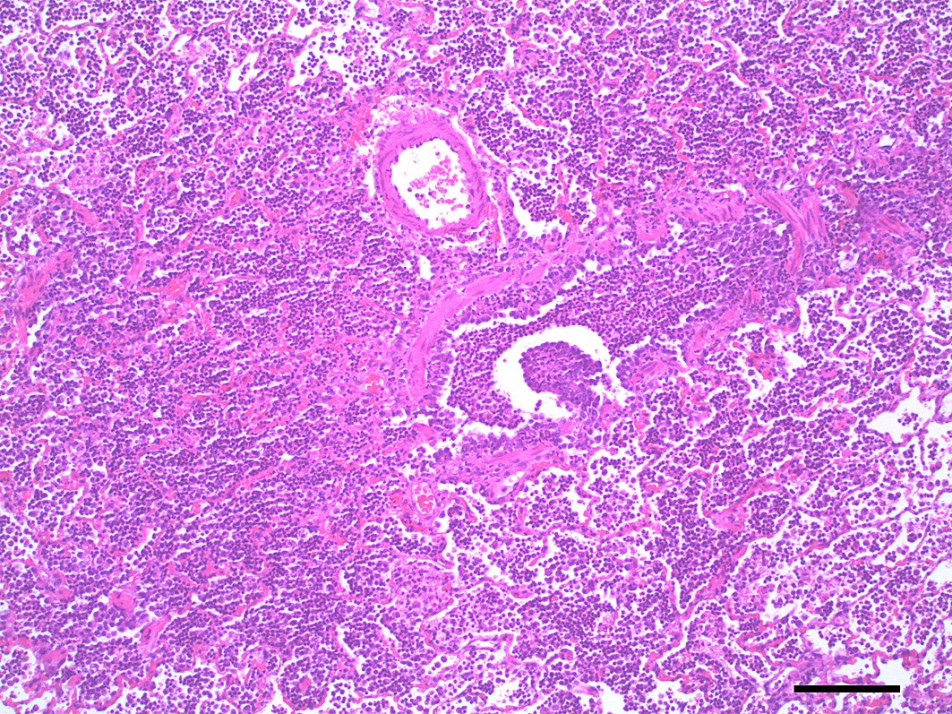
8.1 Isoxazoline acaricides
The development of pharmaceutical isoxazolines formulations that are insecticidal, acaricidal, economical, safe and either topically or orally applied dogs and cats has been a major improvement in the last decade for prevention of tick paralysis. The isoxazolines are potent inhibitors of neurotransmission within insects and ticks (Gassel et al., 2014) and their development resulted from the successful application of genetic technologies and intensive and novel in vitro screening methods (McTier et al., 2016). Pharmacokinetic studies have revealed excellent bioavailability following oral dosing (>85%) and are highly bound to plasma proteins (McTier et al., 2016). The number of isoxazoline containing products approved by the Australian regulator (Australian Pesticides and Veterinary Medicines Authority; APVMA) for control of tick paralysis continues to grow (Table 4). These currently include fluralaner (Gassel et al., 2014), afoxolaner (Shoop et al., 2014), sarolaner (McTier et al., 2016) and lotilaner (Rufener et al., 2017).
Oral administration of fluralaner in dogs was reported as effective against Ixodes holocyclus for at least 115 days and out to 143 days with at least 95% efficacy (Fisara and Webster, 2015). Fluralaner has also been shown to kill adult ticks within 72 h of product administration, although the partially engorged dead ticks may still remain on the dog (Fisara and Webster, 2015). The afoxolaner containing oral product has a minimum duration of action of 30 days and is currently licensed in Australia for control of Ixodes holocyclus infestation in dogs. Oral sarolaner has a claim for 35 days in dogs and has a rapid onset of tick killing (Packianathan et al., 2017). Topical applied and system acting ‘spot-on’ solutions of fluralaner have recently been approved for treatment and control in Australia of Ixodes holocyclus on both cats (Fisara et al., 2018) and dogs. Pharmacokinetic studies of the spot-on formulations of fluralaner in cats and dogs demonstrate a plateau of plasma levels within 3 (cats) and 7 days (dogs), and measurable for at least 12 weeks (Kilp et al., 2016). The estimated systemic bioavailability of the spot-on formulation of fluralaner for both dogs and cats was 25% (Kilp et al., 2016). Efficacy studies of oral lotilaner in dogs against paralysis tick demonstrated at least 87 days duration of action (Baker et al., 2018). Despite the demonstrated effectiveness a general label recommendation is customarily made to end users of all Ixodes holocyclus acaricidal preventatives to thoroughly check animals for ticks daily.
The APVMA provides regulatory guidelines for manufacturers when making new registration applications for Ixodes holocyclus acaricides based on international consensus documents (Curet Bobey, 2015; Marchiondo et al., 2013; Marchiondo et al., 2007). The APVMA recognises the profound implications of live paralysis ticks for dogs and cats. Consequently, data must be generated specifically for each tick species (eg Ixodes holocyclus, Rhipicephalus sanguineus and Haemaphysalis longicornis) a claim is made, products must demonstrate at least 95% efficacy up to 72 hours post-attachment, two dose-confirmation studies must be conducted, studies must use geographically representative ticks, and procedures must be taken to avoid tick transfer between experimental animals during the study periods (eg isolation).
The market for companion animal external parasite control products in healthy and has driven the development of new products such as the isoxazolines. Parasite control products have been reported to represent 18% of the total global animal health market and 46% of the companion animal parasiticide market, with ectoparasiticides representing the largest segment (Curet Bobey, 2015). Australian data published by the APVMA in 2017 suggests that the combined sales of small animal external parasite control products was more than $A160 million per year out of the $A1 billion total veterinary medicines market.
8.2 Other acaricides
Historically, numerous acaricidal preparations have been registered (and deregistered) for the control of paralysis tick in Australia. Fipronil is a topical acaricidal agent that is licensed for control of Ixodes holocyclus on dogs and cats for up to two or three weeks depending upon the formulation used. Fipronil must be applied externally the manufacturer recommends that dogs not be washed or swim within 48 hours of application.
A number of other products have been licensed for control of Ixodes holocyclus in dogs and cats in Australia. Topical skin application of a combination of imidacloprid and permethrin (Advantix, Bayer, Australia) is licensed for control (kills and repels) of paralysis tick for up to two weeks. An insecticidal wash containing pyrethrins, piperonyl butoxide and N-octyl bicycloheptene dicarboximide (Fido's Free-Itch Rinse Concentrate, MavLab, Australia) is licensed for prevention of paralysis tick attachment for up to three days following a single wash. A collar worn by dogs containing flumethrin and propoxur (Kiltix Collar for Dogs, Bayer, Australia) is licensed for control of paralysis tick for 6 weeks. Skin irritation may be a problem for some dogs with prolonged wearing of the collar. The collar manufacturer advises that the collar may lose effectiveness if there is prolonged contact with water. Recently a collar containing imidacloprid and flumethrin has been licensed for use in dogs and cats as a flea and tick preventative (Seresto, Bayer, Australia). The product has a registered claim of 4 months prevention of Ixodes holocyclus infestation. An external skin wash product containing permethrin (25:75 cis:trans) (Permoxin, Dermcare-Vet, Australia) is licensed for control of paralysis tick for seven days. A chemical impregnated collar containing deltamethrin (Scalibor, Intervet, Australia) is licensed for control of paralysis tick in dogs for up to 14 weeks. The manufacturer advises removal of the collar if the dog is swimming in waterways as the active ingredient is toxic to fish and other aquatic life forms. Accidental ingestion of the collar by dogs may result in toxicity with ataxia, drooling and vomiting.
Paralysis tick preventative options for cats were primarily limited to the use of the active ingredient fipronil formulated in either spray and topical products, but recently spot-on isooxazoline has become available (Table 4).
All products are registered as an aid in the control of tick paralysis and do not guarantee complete prevention. All acaricidal products require regular application and lapses with re-treatment of animals may be a primary reason for development of clinical paralysis.
8.3 Development of a toxin vaccine
A key stimulus for funding research into the paralysis tick and its toxins has been to develop an effective vaccine to provide long lasting prevention of the potentially fatal neurotoxic effects in companion and livestock species. Toxoid vaccines against the neurotoxic diseases botulism (Clostridium botulinum) and tetanus (Clostridium tetani) have been highly successful in the livestock and equine industries in Australia and globally. These toxoided vaccines provide long term and highly effective immunity to both diseases. Intuitively the same principles should be applicable to develop of a tick paralysis vaccine. However, it is worth noting that both tetanus and botulism vaccines are not core vaccines for dogs and cats in Australia and their preventative efficacy in these species is largely unknown. The history of tick paralysis vaccine development in Australia is intriguing but to date has not yielded a single registered product.
The long term goal of the work undertaken at CSIRO by the late Dr Bernie Stone in the 1980s was to develop a vaccine for domestic animals in Australia against the paralysis tick neurotoxin (Stone, 1990; Stone et al., 1979). Stone sought to have a one, two or three-shot vaccine that would protect dogs and livestock from the paralysing toxin despite only limited information on the toxin (Stone, 1990). Stone adopted the approach of using salivary gland toxin extracts from ticks engorged on rats and was able to demonstrate protective immunity in the serum of repeatedly immunised rabbits (Stone, 1983) and dogs (Stone et al., 1983). Stone produced a more refined extract from the crude engorged tick preparations, cross linked this with glutaraldehyde, and demonstrated that this toxoided immunogen also resulted in serum that was protective against whole tick toxin extracts (Stone and Neish, 1984). Despite this experimental success the large scale production of a commercial tick vaccine eluded Stone up until the time of his death (Scott, 2005). Arguably the key factor limiting progress to commercialisation would have been producing large enough quantities of toxin from engorged ticks and use of a more animal friendly adjuvant other than Freund’s.
Following on from the preliminary work of Stone a group at the University of Technology in Sydney applied recombinant protein production techniques to the problem of making a tick toxin vaccine (Masina and Broady, 1999). Broady and colleagues produced a recombinant form of holocyclotoxin expressed in E. coli using the nucleotide sequence discovered in their previous work (Thurn et al., 1992). A report was published in 1999 that described production of a recombinant form of the toxin HT-1 and that “…antibodies produced against the recombinant fusion protein show a significant level of protection against native toxins indicating a vaccine is possible…” (Masina and Broady, 1999; Nicholson et al., 2006). Significant homology to scorpion neurotoxins was noted with the nucloetide sequence for HT-1. Chemical synthesis of HT-1 was described more recently along with structural analysis but no data on the biological activity or immunogenicity was published (Vink et al., 2014).
In the most detailed work to date, researchers at the University of Queensland have completed studies on the development of vaccine for dogs (Tabor and Rodriguez-Valle, 2018). In a tantalisingly small vaccine trial, using a novel cocktail consisting of eight recombinant holocyclotoxin peptides, four dogs were successfully protected from developing paralytic effects for 7 days. Three control dogs who did not receive the vaccine developed severe (1 dog), moderate (1 dog) and no (1 dog) signs of tick paralysis (Tabor and Rodriguez-Valle, 2018). Although this data on the effectiveness of dog vaccine is promising further trials are needed to demonstrate longevity of the protective immunity generated in dogs. The duration of immunity following immunisation with a toxoided salivary gland extract was found to be relatively short (Stone et al., 1986). Immunised dogs attained a modest serum neutralising titre after two injections given three weeks apart, but within these declined rapidly and by 22-26 weeks after the last injection the titres had declined to almost that of immunologically naive dogs (Stone et al., 1986). This raises concern about the potential longevity of immunity to an artificial paralysis tick vaccine.
A vaccine would certainly be useful in protecting companion animals and livestock against the paralysing toxin in endemic areas. However, vaccine technology is now competing against the markedly improved range of long acting and relatively economical acaricidal (eg isoxazolines) products for companion animals. Whilst in the 1970s immunological control may have appeared a very attractive control strategy, this is less so in the current era.
8.4 Other preventative methods
Perhaps the best method of preventing tick paralysis is to avoid exposure of susceptible animals by not taking them into endemic tick areas. For a large part of the animal population in Australia tick paralysis will never be an issue because they live permanently outside of these tick areas such as Western Australia. For holidaymakers who travel from non-endemic areas into endemic areas the risk of tick paralysis must be managed (Whitfield et al., 2017).
Regular daily tick searching of animals has been prescribed as a method of early detection of paralysis tick attachment (Ross, 1934). Ross recommended a daily routine of feeling for attached ticks in a dogs coat, rather than relying only on combing and visual searching, and this was a highly effective preventative method in his experience (Ross, 1934). Nevertheless, inexperienced animal owners who attempt this may be at risk of inadvertently not locating a tick and the animal developing fatal paralysis. Researchers who work with ticks and track where they attach can still have difficulty locating all applied ticks, combined with variable locations across the animal’s body this method is probably of low sensitivity for early detection of paralysis ticks.
Tick paralysis continues to present challenges to pets, owners and veterinary practitioners along the east coast of Australia. The enigmatic nature of the neurotoxin has recently been revealed through a long and tortuous pathway of discovery and new preventatives and therapies may evolve from this. There has been a gradual increase in the understanding of the toxin and its mechanism of action. Over 90 years have elapsed since the publication of Sir Ian Clunies Ross’s foundation studies on the tick biology and actions in the dog, but there is still much that is unknown about tick paralysis. Effective preventative treatments are now widely available, but reticence and poor compliance amongst pet owners continues to be a reason to develop ever better clinical treatments and preventatives. Despite the significant amount of research work into a tick toxin vaccine there is currently no commercial product available. The next century of discovery should surely reveal more of the secrets of this much feared, lethal parasite.
Anastopoulos, P., Thurn, M.J., Broady, K.W., 1991. Anticoagulant in the tick Ixodes holocyclus. Australian Veterinary Journal 68, 366-367.
Anon, 2016. Australian Tick Paralysis of Dogs and Cats: Guide to Diagnosis, Management, Treatment and Prevention. Published by Boehringer Ingelheim Animal Health, Australia, Australian Paralysis Tick Advisory Panel.
Atwell, R., Campbell, F., Court, E., 2000. The attachment sites of the paralysis tick (Ixodes holocyclus) on dogs. Aust Vet Practitioner 30, 68-72.
Atwell, R.B., Campbell, F.E., Evans, E.A., 2001. Prospective survey of tick paralysis in dogs. Australian Veterinary Journal 79, 412-418.
Baker, K., Ellenberger, C., Murphy, M., Cavalleri, D., Seewald, W., Drake, J., Nanchen, S., Hacket, K., 2018. Laboratory evaluations of the 3-month efficacy of oral lotilaner (Credelio) against experimental infestations of dogs with the Australian paralysis tick, Ixodes holocyclus. Parasit Vectors 11, 487.
Barker, S.C., Walker, A.R., 2014. Ticks of Australia. The species that infest domestic animals and humans. Zootaxa, 1-144.
Barker, S.C., Walker, A.R., Campelo, D., 2014. A list of the 70 species of Australian ticks; diagnostic guides to and species accounts of Ixodes holocyclus (paralysis tick), Ixodes cornuatus (southern paralysis tick) and Rhipicephalus australis (Australian cattle tick); and consideration of the place of Australia in the evolution of ticks with comments on four controversial ideas. International journal for parasitology 44, 941-953.
Beveridge, I., 1991. Ixodes holocyclus in the Melbourne metropolitan area. Australian Veterinary Journal 68, 214.
Beveridge, I., Coleman, G., 2004. Tick paralysis of dogs in Victoria due to Ixodes cornuatus. Australian Veterinary Journal 82, 642-643.
Broady, K., Thurn, M.J., Masina, S., 1997. Paralysis tick neurotoxin, in: PCT (Ed.).
Brodie, T.G., 1900. The immediate action of an intravenous injection of blood-serum. The Journal of physiology 26, 48-71.
Campbell, F., Atwell, R., 2001. Megaoesophagus in dogs with tick paralysis (Ixodes holocyclus). Aust Vet Practitioner 31, 75-79.
Campbell, F., Atwell, R., 2003. Heart Failure in Dogs With Tick Paralysis Caused by the Australian Paralysis Tick, Ixodes Holocyclus. The International Journal of Applied Research in Veterinary Medicine 1, 148-162.
Campbell, F., Atwell, R., Fenning, A., Hoey, A., Brown, L., 2004. Cardiovascular effects of the toxin(s) of the Australian paralysis tick, Ixodes holocyclus, in the rat. Toxicon 43, 743-750.
Campbell, F.E., Atwell, R.B., 2002. Long QT syndrome in dogs with tick toxicity (Ixodes holocyclus). Australian Veterinary Journal 80, 611-616.
Campbell, F.E., Atwell, R.B., Smart, L., 2003. Effects of the paralysis tick, Ixodes holocyclus, on the electrocardiogram of the Spectacled Flying Fox, Pteropus conspicillatus. Australian Veterinary Journal 81, 328-331.
Chand, K.K., Lee, K.M., Lavidis, N.A., Rodriguez-Valle, M., Ijaz, H., Koehbach, J., Clark, R.J., Lew-Tabor, A., Noakes, P.G., 2016. Tick holocyclotoxins trigger host paralysis by presynaptic inhibition. Scientific reports 6, 29446.
Cooper, B., Cooper, H., Ilkiw, J., Kelly, J., 1976. Tick paralysis, Proceedings No. 30 - Neurology. Sydney Postgraduate Foundation, pp. 57-61.
Cooper, B., Spence, I., 1976. Temperature-dependent inhibition of evoked acetycholine release in tick paralysis. Nature 263, 693-695.
Cortiula, M.W., 1999. Serum and the Soluvac: the Australian approach to whole blood substitutes and blood transfusion during the Second World War. J Hist Med Allied Sci 54, 413-438.
Curet Bobey, M., 2015. Harmonization of regulatory guidelines on efficacy of ectoparasiticides for companion animals: status and missing points. Veterinary parasitology 208, 48-55.
Davey, M., Ratcliffe, R., Titchen, D., 1988. Aspects of the symptomatology and intoxication produced by Ixodes holocyclus. Proceedings of the Sydney Allergen Group 6, 49-.
Day, J., Schull, D., 2008. Findings of the thoracic radiographs of dogs and cats with tick toxicity. Australian Veterinary Practitioner 38, 86-90.
Dodd, S., 1921. Tick Paralysis. Journal of Comparative Pathology and Therapeutics 34, 309-323.
Doube, B., 1975. Cattle and the paralysis tick Ixodes holocyclus. Australian Veterinary Journal 51, 511-515.
Doube, B.M., Goodger, B.V., Stone, B.F., Nolan, J., 1995. Toxic secretions in cuticular washings from the Australian paralysis tick, Ixodes holocyclus. Toxicon 33, 304.
Fearnley, A., 2002. The importance of body temperature in the treatment of tick paralysis. Aust Vet Practitioner 32, 137-138.
Fisara, P., Guerino, F., Sun, F., 2018. Investigation of the efficacy of fluralaner spot-on (Bravecto(R)) against infestations of Ixodes holocyclus on cats. Parasit Vectors 11, 366.
Fisara, P., Webster, M., 2015. A randomized controlled trial of the efficacy of orally administered fluralaner (Bravecto) against induced Ixodes holocyclus (Australian paralysis tick) infestations on dogs. Parasit Vectors 8, 257.
Fitzgerald, M., 1998. Ixodes holocyclus poisoning, Clinical Toxicology, Sydney Postgraduate Foundation, pp. 203-220.
Fitzgerald, M., 2007. Tick toxicity in a cat caused by the larval stage of Ixodes holocyclus. Aust Vet Practitioner 37, 32.
Gassel, M., Wolf, C., Noack, S., Williams, H., Ilg, T., 2014. The novel isoxazoline ectoparasiticide fluralaner: selective inhibition of arthropod gamma-aminobutyric acid- and L-glutamate-gated chloride channels and insecticidal/acaricidal activity. Insect Biochem Mol Biol 45, 111-124.
Gerasimova, M., Kelman, M., Ward, M.P., 2018. Are recreational areas a risk factor for tick paralysis in urban environments? Veterinary parasitology 254, 72-77.
Goodrich, B.S., Murray, M.D., 1978. Factors influencing the toxicity of salivary gland extracts of Ixodes holocyclus Neumann. International journal for parasitology 8, 313-320.
Hall-Mendelin, S., Craig, S.B., Hall, R.A., O'Donoghue, P., Atwell, R.B., Tulsiani, S.M., Graham, G.C., 2011a. Tick paralysis in Australia caused by Ixodes holocyclus Neumann. Annals of tropical medicine and parasitology 105, 95-106.
Hall-Mendelin, S., O'Donoghue, P., Atwell, R.B., Lee, R., Hall, R.A., 2011b. An ELISA to Detect Serum Antibodies to the Salivary Gland Toxin of Ixodes holocyclus Neumann in Dogs and Rodents. Journal of parasitology research 2011, 283416.
Hamilton, D., 1940. Tick paralysis: a dangerous disease in children. Medical Journal Australia 22, 759-765.
Hindmarsh, W., Pursell, R., 1935. Tick paralysis of dogs. Mortality after serum treatment. Australian Veterinary Journal, 229-234.
Holland, C.T., 2008. Asymmetrical focal neurological deficits in dogs and cats with naturally occurring tick paralysis (Ixodes holocyclus): 27 cases (1999-2006). Australian Veterinary Journal 86, 377-384.
Hutton, J.B., 1974. Tick (Ixodes holocyclus) paralysis in an imported dog. New Zealand veterinary journal 22, 173.
Ilkiw, J.E., 1983. Tick paralysis in Australia, in: Kirk (Ed.), Current Veterinary Therapy IX. Lea and Febiger.
Ilkiw, J.E., Turner, D.M., 1988. Infestation in the dog by the paralysis tick, Ixodes holocyclus. 5. Treatment. Australian Veterinary Journal 65, 236-238.
Ilkiw, J.E., Turner, D.M., Howlett, C.R., 1987. Infestation in the dog by the paralysis tick Ixodes holocyclus. 1. Clinical and histological findings. Australian Veterinary Journal 64, 137-139.
Isbister, G.K., Bawaskar, H.S., 2014. Scorpion envenomation. The New England journal of medicine 371, 457-463.
Jackson, J., Beveridge, I., Chilton, N.B., Andrews, R.H., 2007. Distributions of the paralysis ticks Ixodes cornuatus and Ixodes holocyclus in south-eastern Australia. Australian Veterinary Journal 85, 420-424.
Jones, D., 1991. Tick paralysis, Proceedings No. 149 - Emergency medicine and critical care. Sydney Postgraduate Foundation., pp. 153-165.
Jones, R.M., Kalman, S.M., 1980. Cardioactive substances in blood: the actions of serotonin on isolated guinea-pig atria. Gen Pharmacol 11, 463-467.
Kaire, G., 1965. A method for assay of canine tick anti-serum. Australian Veterinary Journal 41, 239-240.
Kaire, G.H., 1966. Isolation of tick paralysis toxin from Ixodes holocyclus. Toxicon 4, 91-97.
Karbanowicz, T., Dover, E., Mu, X., Tabor, A., Rodriguez-Valle, M., 2017. Extracellular expression of the HT1 neurotoxin from the Australian paralysis tick in two Saccharomyces cerevisiae strains. Toxicon 140, 1-10.
Kelers, K., Barker, S., Song, S., 2012. Identification of Ixodes holocyclus by DNA sequencing: application for suspected tick envenomation in a dog living outside an enzootic area. Australian Veterinary Practitioner 42, 240-.
Kilp, S., Ramirez, D., Allan, M.J., Roepke, R.K., 2016. Comparative pharmacokinetics of fluralaner in dogs and cats following single topical or intravenous administration. Parasit Vectors 9, 296.
Kwak, M.L., 2017. Keys for the morphological identification of the Australian paralysis ticks (Acari: Ixodidae), with scanning electron micrographs. Experimental & applied acarology 72, 93-101.
Leister, E., Morton, J., Atwell, R., Webster, R., 2018. Clinical presentations, treatments and risk factors for mortality in cats with tick paralysis caused by Ixodes holocyclus: 2077 cases (2008-2016). Journal of Feline Medicine and Surgery 20, 465-478.
Lydecker, H.W., Stanfield, E., Lo, N., Hochuli, D.F., Banks, P.B., 2015. Are urban bandicoots solely to blame for tick concerns? Australian Zoologist 37, 288-293.
Malik, R., Farrow, B.R., 1991. Tick paralysis in North America and Australia. The Veterinary Clinics of North America. Small Animal Practice 21, 157-171.
Malik, R., King, J., Allan, G., 1988. Megaoesophagus associated with tick paralysis in three dogs. Aust Vet Practitioner 18, 156-159.
Marchiondo, A.A., Holdsworth, P.A., Fourie, L.J., Rugg, D., Hellmann, K., Snyder, D.E., Dryden, M.W., World Association for the Advancement of Veterinary, P., 2013. World Association for the Advancement of Veterinary Parasitology (W.A.A.V.P.) second edition: guidelines for evaluating the efficacy of parasiticides for the treatment, prevention and control of flea and tick infestations on dogs and cats. Veterinary parasitology 194, 84-97.
Marchiondo, A.A., Holdsworth, P.A., Green, P., Blagburn, B.L., Jacobs, D.E., 2007. World Association for the Advancement of Veterinary Parasitology (W.A.A.V.P.) guidelines for evaluating the efficacy of parasiticides for the treatment, prevention and control of flea and tick infestation on dogs and cats. Veterinary parasitology 145, 332-344.
Masina, S., Broady, K.W., 1999. Tick paralysis: development of a vaccine. International journal for parasitology 29, 535-541.
McTier, T.L., Chubb, N., Curtis, M.P., Hedges, L., Inskeep, G.A., Knauer, C.S., Menon, S., Mills, B., Pullins, A., Zinser, E., Woods, D.J., Meeus, P., 2016. Discovery of sarolaner: A novel, orally administered, broad-spectrum, isoxazoline ectoparasiticide for dogs. Veterinary parasitology 222, 3-11.
Murray, M., Koch, J., 1969. The engorgement of Ixodes holocyclus Neuman (Acarina: Ixodoidea) on mice. J Aust Ent Soc 8, 187-.
Nicholson, G.M., Graudins, A., Wilson, H.I., Little, M., Broady, K.W., 2006. Arachnid toxinology in Australia: from clinical toxicology to potential applications. Toxicon 48, 872-898.
Nicolson, G.P., McGrath, A., Webster, R.A., Li, J., Kaye, S., Malik, R., Beijerink, N.J., 2016. NT-proBNP and cardiac troponin I concentrations in dogs with tick paralysis caused by Ixodes holocyclus. Australian Veterinary Journal 94, 274-279.
Ong, C., Rodriguez-Valle, M., Moolhuijzen, P., Barrero, R.A., Hunter, A., Szabo, T., Bellgard, M., Lew-Tabor, A., 2016. Exploring the transcriptomic data of the Australian paralysis tick, Ixodes holocyclus. Journal of Veterinary Science 3.
Oxer, D., 1948. The preparation of canine anti-tick serum. Australian Veterinary Journal, 95-96.
Oxer, D., Ricardo, C., 1942. Notes on the biology, toxicity and breeding of Ixodes holocyclus (Neuman). Australian Veterinary Journal 18, 194-199.
Packianathan, R., Hodge, A., Bruellke, N., Davis, K., Maeder, S., 2017. Comparative speed of kill of sarolaner (Simparica(R)) and afoxolaner (NexGard(R)) against induced infestations of Ixodes holocyclus on dogs. Parasit Vectors 10, 98.
Padula, A.M., 2016. Tick Paralysis of Animals in Australia, in: Gopalakrishnakone, P., Faiz, S.M.A., Gnanathasan, C.A., Habib, A.G., Fernando, R., Yang, C.-C., Vogel, C.-W., Tambourgi, D.V., Seifert, S.A. (Eds.), Clinical Toxinology: Clinical Toxinology. Springer Netherlands, Dordrecht, pp. 1-20.
Padula, A.M., Winkel, K.D., 2016. Fatal presumed tiger snake (Notechis scutatus) envenomation in a cat with measurement of venom and antivenom concentration. Toxicon 113, 7-10.
Pearn, J., 1977. Neuromuscular paralysis caused by tick envenomation. Journal of the neurological sciences 34, 37-42.
Reid, G., Bick, M., 1942. Pharmacologically active substances in serum. Aust J Exp Biol Med Sci 20, 33-46.
Riek, R., 1957. Studies on the reactions of animals to infestation with ticks II. Tick Toxins. Aust J Agric Res 8, 215-.
Rodriguez-Valle, M., Moolhuijzen, P., Barrero, R.A., Ong, C.T., Busch, G., Karbanowicz, T., Booth, M., Clark, R., Koehbach, J., Ijaz, H., Broady, K., Agnew, K., Knowles, A.G., Bellgard, M.I., Tabor, A.E., 2018. Transcriptome and toxin family analysis of the paralysis tick, Ixodes holocyclus. International journal for parasitology 48, 71-82.
Ross, I., 1924. The bionomics of Ixodes holocyclus Neumann, with a redescription of the adult and nymphal stages and a description of the larvae. Parasitology 16, 365.
Ross, I., 1926. An experimental study of tick paralysis in Australia. Parasitology 18, 410-.
Ross, I., 1927a. An experimental study of tick paralysis in Australia. Australian Veterinary Journal, 71-72.
Ross, I., 1927b. The Treatment of Tick Paralysis. Australian Veterinary Journal 3, 72-74.
Ross, I., 1932. Tick paralysis in the dog caused by nymphs of Ixodes holocyclus. Australian Veterinary Journal 8, 102-104.
Ross, I., 1934. Tick paralysis in the dog. Period elapsing between attachment of tick and the onset of symptoms. Australian Veterinary Journal 10, 182-183.
Ross, I., 1935. Tick paralysis: a fatal disease of dogs and other animals in Eastern Australia. Journal of the Council for Scientific and Industrial Research 8, 8-13.
Rufener, L., Danelli, V., Bertrand, D., Sager, H., 2017. The novel isoxazoline ectoparasiticide lotilaner (Credelio): a non-competitive antagonist specific to invertebrates gamma-aminobutyric acid-gated chloride channels (GABACls). Parasit Vectors 10, 530.
Ryan, A., 1952. Tick paralysis in Tasmania. Australian Veterinary Journal, 247.
Schull, D., 2007. Acute side effects attributed to the use of tick antitoxin serum: a review of available descriptions. Aust Vet Practitioner 37, 98.
Schull, D., 2008. The use of tick antitoxin serum (TAS) and associated drug therapies for the management of Ixodes holocyclus toxicity in dogs.
Schull, D.N., Litster, A.L., Atwell, R.B., 2007. Tick toxicity in cats caused by Ixodes species in Australia: a review of published literature. Journal of Feline Medicine and Surgery 9, 487-493.
Scott, A., 2005. Dr Bernard Stone AM, DSc. Australian Society for Parasitology Newsletter 16, 4-5.
Shoop, W.L., Hartline, E.J., Gould, B.R., Waddell, M.E., McDowell, R.G., Kinney, J.B., Lahm, G.P., Long, J.K., Xu, M., Wagerle, T., Jones, G.S., Dietrich, R.F., Cordova, D., Schroeder, M.E., Rhoades, D.F., Benner, E.A., Confalone, P.N., 2014. Discovery and mode of action of afoxolaner, a new isoxazoline parasiticide for dogs. Veterinary parasitology 201, 179-189.
Stone, B., 1983. Vaccination of rabbits against the toxin of the Australian paralysis tick Ixodes holocyclus. Toxicon, 421-424.
Stone, B., 1990. No.3 : Tick paralysis: Antitoxin and vaccine. Sydney Postgraduate Foundation. Perspectives, Mailing No. 153, Perspective No. 3, pp 9-12, Apr 1990. 9-12.
Stone, B., Doube, B., Binnington, K., 1979. Toxins of the Australian paralysis tick Ixodes holcyclus. Recent Advances in Acarology 1, 347-.
Stone, B., JH, A., 1987. Tick toxicoses and the causal toxins: tick paralysis, in: Gopalakrishnakone, P., Tan, C. (Eds.), Progress in venom and toxin research, Singapore, pp. 594-.
Stone, B., Wright, I., 1981. Tick toxins and protective immunity, in: Whitehead, G., Gibson, J. (Eds.), Tick Biology and Control, Grahamstown, South Africa, pp. 1-5.
Stone, B., Wright, I., Neish, A.L., 1983. Natural and artificial immunisation of dogs against tick paralysis induced by the Australian paralysis tick Ixodes holocyclus. Toxicon, 417-420.
Stone, B., Wright, I.G., 1979. Toxins of Ixodes holocyclus and immunity to paralysis, Annual Conference of the Australian Veterinary Association, Townsville, pp. 75-78.
Stone, B.F., Cowie, M.R., Kerr, J.D., Binnington, K.C., 1982. Improved toxin/antitoxin assays for studies on the Australian paralysis tick Ixodes holocyclus. Aust J Exp Biol Med Sci 60, 309-318.
Stone, B.F., Neish, A.L., 1984. Tick-paralysis toxoid: an effective immunizing agent against the toxin of Ixodes holocyclus. Aust J Exp Biol Med Sci 62 ( Pt 2), 189-191.
Stone, B.F., Neish, A.L., Morrison, J.J., Uren, M.F., 1986. Toxoid stimulation in dogs of high titres of neutralising antibodies against holocyclotoxin, the paralysing toxin of the Australian paralysis tick Ixodes holocyclus. Australian Veterinary Journal 63, 125-127.
Tabor, A., Rodriguez-Valle, M., 2018. New vaccine protects against paralysis tick, University of Qld.
Thurn, M.J., Gooley, A., Broady, K., 1992. Identification of the neurotoxin from the Australian paralysis tick, Ixodes holocyclus, in: Gopalakrishnakone, P., Tan, C. (Eds.), Recent Advances in Toxinology research, Singapore, pp. 243-265.
Trigg, N., Leister, E., Whitney, J., McAlees, T., 2014. Outcomes of mechanical ventilation in 302 dogs and cats in Australia (2005-2013). Aust Vet Practitioner 44, 698-703.
Vink, S., Daly, N.L., Steen, N., Craik, D.J., Alewood, P.F., 2014. Holocyclotoxin-1, a cystine knot toxin from Ixodes holocyclus. Toxicon 90, 308-317.
Webster, R.A., 2014a. Tick Paralysis 1: Pathophysiology, Controversies And Initial Management, Continuing Veterinary Education, Emergency Conference, Proceedings No. 411.
Webster, R.A., 2014b. Tick Paralysis 2: Critical Care Of Patients With Tick Paralysis, Continuing Veterinary Education, Proceedings No. 411, p. 91.
Webster, R.A., Haskins, S., Mackay, B., 2013a. Management of respiratory failure from tick paralysis. Australian Veterinary Journal 91, 499-504.
Webster, R.A., Mackie, J.T., Haskins, S.C., 2013b. Histopathological changes in the lungs from dogs with tick paralysis: 25 cases (2010-2012). Australian Veterinary Journal 91, 306-311.
Webster, R.A., Mills, P.C., Morton, J.M., 2013c. Indications, durations and outcomes of mechanical ventilation in dogs and cats with tick paralysis caused by Ixodes holocyclus: 61 cases (2008-2011). Australian Veterinary Journal 91, 233-239.
Westwood, M.N., Emery, D.L., Dhand, N.K., 2013. Clinical presentation and treatment of tick paralysis in dogs and cats in Sydney (2001-2010). Australian Veterinary Journal 91, 491-498.
Whitfield, Z., Kelman, M., Ward, M.P., 2017. Delineation of an endemic tick paralysis zone in southeastern Australia. Veterinary parasitology 247, 42-48.
Zucker, I., Cornish, K., 1981. The Bezold-Jarisch Reflex in the Conscious Dog. Circ Res 49, 940-948.